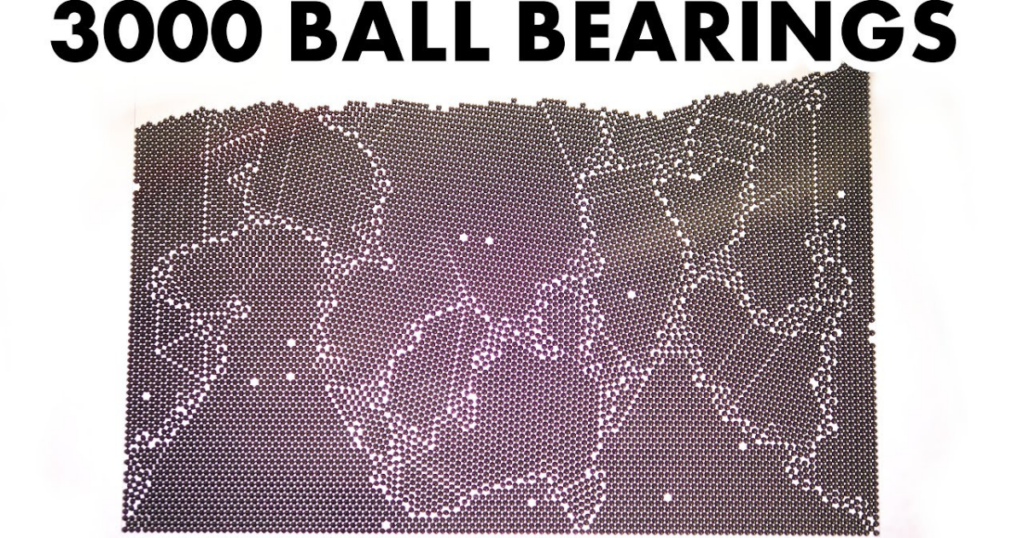
Have you ever wondered how heating metal affects its properties? This article explores this fascinating topic using a clever, homemade model called “Atomic”. We’ll delve into the connection between atomic jiggling, grain size, and the surprising impact it has on a metal’s ability to bend without breaking.
The Model: A Playground for Atoms
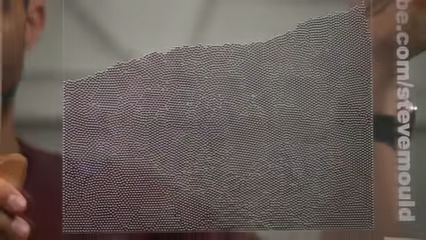
The Atomic model consists of numerous ball bearings trapped between two clear plates. These balls represent atoms in a metal lattice, free to move and naturally arrange themselves into a regular pattern. However, imperfections within the lattice mimic the real world, where metals have flaws in their atomic structure.
The Experiment: Simulating Heat Treatment
Initially, the balls settle into small regions of order, resembling the crystalline structure of metals. These regions, called grains, have varying orientations and cannot merge into a single, perfect crystal. The size of these grains significantly impacts the metal’s physical properties, particularly its strength and ductility .
To explore how heat treatment affects grain size, we simulate the process by “jiggling” the balls using a vibrating device. This added kinetic energy mimics the increased thermal energy (heat) experienced by atoms in a heated metal. As a result, the balls rearrange, forming larger grains.
The Counterintuitive Outcome: Bigger Grains, Easier Bending
One might expect larger, more organized grains to make the metal stronger. However, the surprising truth is that larger grains make the metal easier to bend permanently, or more “ductile.” This might seem counterintuitive, but the answer lies in the world of imperfections called “dislocations.”
Dislocations: The Culprit Behind Bending
Dislocations are imperfections within the crystal structure, resembling an extra half-plane of atoms inserted into the lattice. These imperfections, while seemingly detrimental, are surprisingly easy to move due to the strained bonds surrounding them.
When you apply force to a metal, these dislocations move in various directions. Importantly, it takes less energy to move dislocations within a single grain than to move entire grains against each other. Dislocations essentially act as the “carriers of plasticity” in a metal, allowing it to bend and deform.
The Grain Boundary Barrier: Why Smaller Grains Resist Bending
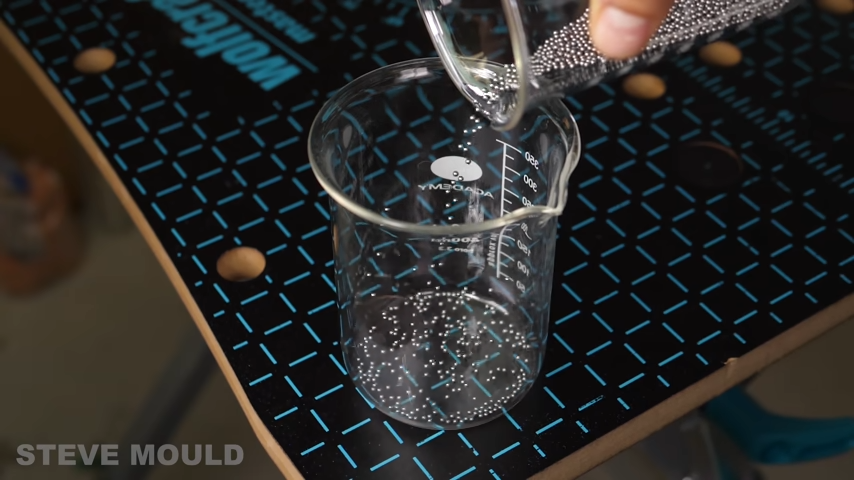
Smaller grains have a crucial advantage when it comes to resisting bending. In a metal with small grains, dislocations within a grain encounter grain boundaries (the borders between grains) much sooner. These boundaries act as barriers, stopping the dislocations from traveling further and hindering the metal’s ability to deform permanently.
On the other hand, larger grains offer more “real estate” for dislocations to move, allowing them to travel further before reaching a grain boundary. This essentially increases the metal’s capacity to bend before breaking, making it more ductile.
Beyond the Model: Exploring Further
While the Atomic model provides a simplified yet insightful glimpse into the world of metallurgy, it’s important to acknowledge its limitations. Various heat treatment techniques like annealing, tempering, and quenching exist, each with its own effects on the metal’s properties. Additionally, factors like temperature, cooling rate, and the presence of other elements influence the final outcome.
This exploration, however, highlights the fascinating interplay between atomic structure, grain size, and dislocations, offering a deeper understanding of how seemingly simple actions like heating a metal can significantly alter its strength and ductility.